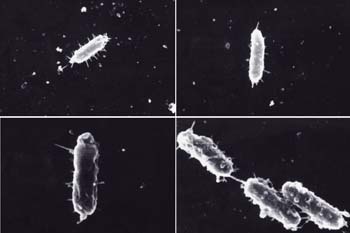
“ropes” called fimbrae that are used to attach to surfaces.
Mark Schneegurt studied how these “stuck” bacteria interact
with metals.
Soil contamination by hazardous substances affects plants, animals and humans alike as pollutants travel up the food chain.
Current methods of cleanup, according to the EPA, include isolation, incineration or the addition of more chemicals. These are large fixes for a very small problem — that is, the problem of the wrong atoms in the wrong place.
Researchers know heavy metals such as chromium, molybdenum, lead and arsenic don’t move through groundwater unassisted — bacteria are involved.
Mark Schneegurt, an assistant professor in WSU’s biological sciences department, explains that scientists aren’t sure whether bacteria help or hinder the metals’ travel: “Probably both,” he admits.
In theory, molecules hitch a ride on free-floating bacteria; bacteria stuck to sand or other objects, however, catch metals and fix them in place. How this happens and where on the bacterium — inside or outside in the many layers of “skin” around the cell membrane — the toxic particles bind is difficult to measure. That’s why, when Schneegurt and colleagues at the University of Chicago created a process to do just that, it merited a write-up in the prestigious Science magazine in Oct. 2004.
Turns out the most powerful X-rays in the solar system are located in Chicago, at the Argonne National Laboratory. The Advanced Photon Source, says Schneegurt, is “a big toy,” a multimillion-dollar particle accelerator with only one rival in the world. “It’s been known to set off Geiger counters in the parking lot,” boasts Schneegurt.
The energy produced is so vast that the lead-walled room the beam shines into “wouldn’t stop the beam, but would give you time to shut it off if a whole lot of things went wrong.” The massive machine emits full-spectrum radiation, but Schneegurt’s team pared this down to X-rays alone — important because these rays give off no heat — and focused it using Fresnel lenses to an infinitesimal 150 nanometers.
This tiny beam was shot at living bacteria mixed with chromium; in a process similar to rippling in a pond, the radiation bounces off the metal, and the pattern of waves recorded, called XRF microscopy, shows what substances are involved, what state they’re in and who’s their nearest neighbor — exactly what’s necessary to study how metals bind to bacteria.
“What makes this technique novel,” says Schneegurt, is the third-generation synchotron facility, which produces energy far beyond what previous researchers have been able to use. Also revolutionary is their use of living cells, which amazingly survived the process. “It’s a fabulous technique,” Schneegurt says. “Nothing like this has ever been done before.”
Preliminary findings show that the bond between metals and free-floating bacteria is different from that between metals and “stuck” bacteria, and that the metals seem to fasten to the extracellular slime on the outside of the organism.
Soil contamination is only one field affected by these experiments. Schneegurt’s excited about a far less earthly application: finding life in outer space. “Life leaves a signature,” he stresses.
A rock brought back from Mars can’t be examined in traditional ways for signs of microbes; but Schneegurt’s non-invasive technique may be useful for reading biology’s handwriting — a telltale scrawl called the Redox Tower, unique to living things. So in the future, it may be reactions at the smallest level that resound throughout the huge question of our place in the universe.